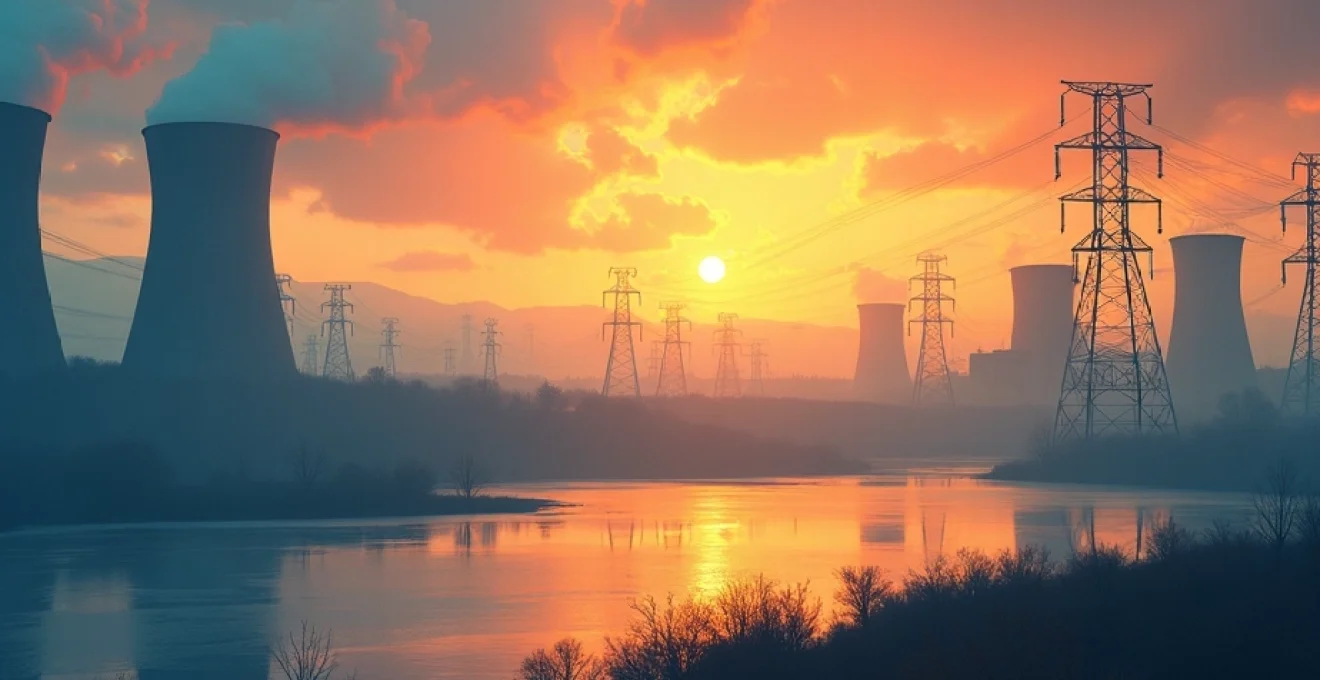
As the world grapples with the urgent need to reduce greenhouse gas emissions, nuclear energy emerges as a powerful ally in the transition to a low-carbon future. This clean, reliable, and efficient power source has the potential to play a crucial role in meeting global energy demands while minimizing environmental impact. By harnessing the power of the atom, nations can significantly reduce their carbon footprint and pave the way for a more sustainable energy landscape.
Nuclear fission technology in low-carbon power generation
Nuclear fission, the process that powers current nuclear reactors, has been a cornerstone of low-carbon electricity production for decades. This technology splits uranium atoms to release enormous amounts of energy, which is then converted into electricity. The efficiency of nuclear fission is remarkable, with a single uranium fuel pellet containing as much energy as one ton of coal or 149 gallons of oil.
Modern nuclear power plants have evolved to become incredibly efficient and safe. They operate at capacity factors exceeding 90%, meaning they produce maximum power for more than 90% of the time annually. This reliability far surpasses that of fossil fuel plants and intermittent renewables, making nuclear an ideal baseload power source.
The carbon footprint of nuclear energy is minimal, with lifecycle emissions comparable to those of wind power. According to the Intergovernmental Panel on Climate Change (IPCC), nuclear energy produces a median of 12 grams of CO2 equivalent per kilowatt-hour, which is about 1/40th the carbon intensity of natural gas and 1/80th that of coal.
Nuclear power plants provide a stable, low-carbon energy source that can operate continuously, regardless of weather conditions or time of day.
As countries strive to meet ambitious climate targets, many are reconsidering nuclear energy as a vital component of their energy mix. France, for example, generates about 70% of its electricity from nuclear power, boasting one of the lowest carbon intensities among developed nations.
Advanced reactor designs for enhanced safety and efficiency
The nuclear industry is not resting on its laurels. Significant advancements in reactor design are pushing the boundaries of safety, efficiency, and versatility. These innovations aim to address public concerns, reduce costs, and expand the applications of nuclear technology.
Small modular reactors (SMRs): NuScale and Rolls-Royce models
Small Modular Reactors (SMRs) represent a revolutionary approach to nuclear power generation. These compact, factory-built reactors can be transported to sites for assembly, offering flexibility and scalability that traditional large-scale reactors cannot match. NuScale Power, a leading SMR developer, has designed a 77 MWe module that can be combined to create power plants of various sizes.
Rolls-Royce is also entering the SMR market with its UK SMR design, aiming to deliver 470 MWe of power from a single unit. These SMRs promise reduced construction times, lower capital costs, and enhanced safety features, making nuclear power more accessible to a broader range of communities and applications.
Generation IV reactors: molten salt and fast neutron systems
Generation IV reactors are at the forefront of nuclear innovation, offering improved safety, efficiency, and waste management. Molten salt reactors (MSRs) use liquid fuel, which can operate at higher temperatures and lower pressures than traditional solid-fuel reactors. This design inherently reduces the risk of meltdowns and allows for more efficient power generation.
Fast neutron reactors, another Generation IV technology, can utilize nuclear waste as fuel, significantly reducing the volume and radiotoxicity of waste while extracting more energy from uranium resources. These reactors have the potential to close the nuclear fuel cycle, addressing one of the primary concerns about nuclear energy.
Fusion reactor prototypes: ITER and commonwealth fusion systems
While nuclear fission has been the mainstay of nuclear power, fusion technology promises an even cleaner and more abundant energy source. The International Thermonuclear Experimental Reactor (ITER) project, a collaboration of 35 nations, is constructing the world's largest tokamak fusion device in southern France. ITER aims to demonstrate the feasibility of fusion as a large-scale, carbon-free energy source.
Private companies like Commonwealth Fusion Systems are also making strides in fusion technology. Their SPARC reactor design utilizes high-temperature superconducting magnets to achieve fusion in a more compact and potentially commercially viable device. If successful, fusion could provide virtually limitless clean energy with minimal radioactive waste.
Accident-tolerant fuel development: westinghouse EnCore fuel
Enhancing the safety and performance of nuclear fuel is a critical area of research. Westinghouse Electric Company has developed EnCore Fuel, an accident-tolerant fuel designed to withstand severe accident conditions for longer periods. This innovative fuel features a silicon carbide cladding and uranium silicide pellets, offering improved heat tolerance and reduced hydrogen generation during high-temperature steam exposure.
The implementation of accident-tolerant fuels like EnCore could significantly enhance the safety margins of existing and future nuclear reactors, addressing public concerns and potentially extending the operational lifetime of nuclear plants.
Nuclear waste management and sustainable fuel cycles
Addressing nuclear waste management is crucial for the long-term sustainability of nuclear energy. Innovative approaches to waste reduction, storage, and recycling are being developed to minimize environmental impact and maximize resource utilization.
Deep geological repositories: Finland's Onkalo project
Finland is leading the way in long-term nuclear waste storage with its Onkalo deep geological repository. Located 400-450 meters underground, this facility is designed to safely store spent nuclear fuel for up to 100,000 years. The repository utilizes multiple engineered and natural barriers to isolate the waste from the environment.
The success of the Onkalo project could serve as a model for other nations seeking long-term solutions for nuclear waste storage. Similar repositories are being planned or constructed in Sweden, France, and other countries with significant nuclear power programs.
Closed fuel cycle technologies: pyrochemical processing
Closed fuel cycle technologies aim to recycle spent nuclear fuel, reducing waste volume and extracting additional energy from used fuel. Pyrochemical processing, also known as pyroprocessing, is an advanced method for separating and recycling nuclear materials.
This technique uses high-temperature molten salts to separate uranium and transuranic elements from fission products. The recovered materials can then be fabricated into new fuel for fast neutron reactors, significantly reducing the volume and radiotoxicity of nuclear waste while extending fuel resources.
Transmutation of long-lived radionuclides
Transmutation is an innovative approach to dealing with long-lived radioactive isotopes in nuclear waste. This process involves converting these isotopes into shorter-lived or stable elements through nuclear reactions. Accelerator-driven systems (ADS) and fast neutron reactors can be used for transmutation, potentially reducing the required isolation time for nuclear waste from hundreds of thousands of years to just a few hundred years.
Advanced waste management technologies have the potential to significantly reduce the environmental footprint of nuclear energy and address public concerns about long-term waste storage.
Integration of nuclear power in renewable energy grids
As the world transitions to cleaner energy sources, the integration of nuclear power with renewable energy systems becomes increasingly important. Nuclear energy can provide the stable baseload power needed to complement the intermittent nature of wind and solar generation.
Load-following capabilities of modern nuclear plants
Modern nuclear reactors are designed with enhanced load-following capabilities, allowing them to adjust their output in response to grid demands. This flexibility enables nuclear plants to work in harmony with variable renewable sources, ramping up production when wind or solar output decreases and reducing output during periods of high renewable generation.
France's nuclear fleet demonstrates this capability, with many reactors routinely operating in load-following mode. This flexibility not only supports grid stability but also enhances the economic competitiveness of nuclear power in energy markets with high renewable penetration.
Hybrid Nuclear-Renewable systems: NREL's NICE future initiative
The National Renewable Energy Laboratory (NREL) is spearheading the Nuclear Innovation: Clean Energy (NICE) Future initiative, which explores synergies between nuclear and renewable energy systems. These hybrid systems could maximize clean energy production while minimizing curtailment and storage requirements.
One concept under investigation is the use of nuclear heat for thermal energy storage, allowing excess energy to be stored during low demand periods and released when needed. This approach could significantly enhance grid flexibility and reliability in a low-carbon energy system.
Grid stability enhancement through nuclear baseload
Nuclear power's ability to provide consistent, large-scale baseload electricity is crucial for maintaining grid stability as the share of variable renewable energy increases. The inertia provided by nuclear generators helps to stabilize grid frequency and voltage, reducing the risk of blackouts and improving overall power quality.
By providing this stable foundation, nuclear energy enables higher penetration of renewable sources without compromising grid reliability. This synergy between nuclear and renewables is essential for achieving deep decarbonization of electricity systems.
Economic and policy frameworks for nuclear energy expansion
The future of nuclear energy in a low-carbon world depends not only on technological advancements but also on supportive economic and policy frameworks. Addressing the high upfront costs of nuclear projects and creating a level playing field for all low-carbon technologies are crucial steps in realizing nuclear's potential.
Levelized cost of electricity (LCOE) analysis for nuclear vs. alternatives
When evaluating the economic competitiveness of nuclear energy, it's essential to consider the Levelized Cost of Electricity (LCOE). This metric accounts for the total cost of building and operating a power plant over its lifetime, divided by the total electricity output. While nuclear power has high upfront capital costs, its low fuel and operating costs can make it competitive over the long term.
Recent LCOE analyses show that nuclear power can be cost-competitive with other low-carbon technologies, especially when considering the system costs of integrating variable renewable sources. However, the competitiveness of nuclear varies significantly based on factors such as construction costs, financing terms, and policy support.
Carbon pricing mechanisms and nuclear competitiveness
Carbon pricing mechanisms, such as carbon taxes or cap-and-trade systems, can significantly enhance the competitiveness of nuclear power relative to fossil fuel generation. By internalizing the environmental costs of carbon emissions, these policies create a more level playing field for low-carbon technologies.
Countries with robust carbon pricing schemes have seen increased interest in nuclear energy as a means of meeting emission reduction targets while maintaining reliable electricity supply. For example, the UK's Carbon Price Floor has contributed to the economic case for new nuclear projects like Hinkley Point C.
International cooperation: IAEA's milestones approach for nuclear infrastructure
The International Atomic Energy Agency (IAEA) has developed the Milestones Approach to assist countries in developing the necessary infrastructure for a nuclear power program. This comprehensive framework covers 19 infrastructure issues, including legal and regulatory frameworks, human resource development, and stakeholder involvement.
By following the Milestones Approach, countries can ensure a systematic and thorough preparation for introducing nuclear power, addressing key challenges and building the capacity needed for safe and successful implementation. This international cooperation is crucial for expanding nuclear energy's role in a low-carbon future, particularly in emerging economies.
As the world grapples with the dual challenges of meeting growing energy demands and mitigating climate change, nuclear energy stands out as a powerful tool in our clean energy arsenal. Through continued innovation, responsible waste management, and thoughtful integration with renewable sources, nuclear power can play a pivotal role in shaping a sustainable, low-carbon future for generations to come.